As a first-year professor at Georgia Tech, I have spent the past two semesters engaged in the exciting process of building a research group. The first few months after I arrived were a whirlwind – supplies were purchased, equipment was built, and we moved into our lab. We were then able to jump into research […]
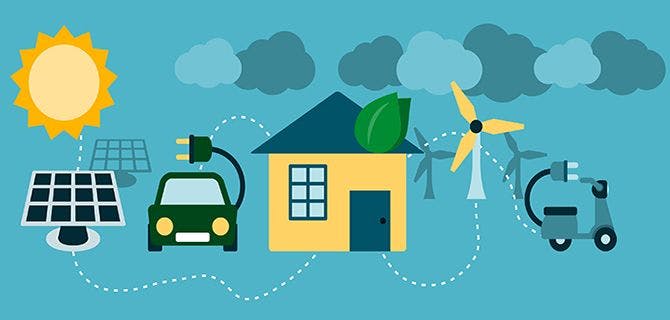
As a first-year professor at Georgia Tech, I have spent the past two semesters engaged in the exciting process of building a research group. The first few months after I arrived were a whirlwind – supplies were purchased, equipment was built, and we moved into our lab. We were then able to jump into research and have since started a number of projects. My group’s research goal is to transform the scientific community’s understanding of how materials inside energy devices change, transform, and degrade during operation. Such knowledge is the first step in engineering new and improved energy technologies, such as high-energy batteries or devices that produce fuel from sunlight, that will accelerate our society’s shift to clean energy.
Over the past two decades, scientists and engineers have made significant advances towards controlling and understanding the structure, chemistry, and properties of materials at the nanoscale. However, to use engineered nanomaterials in applications, knowledge of the initial, static structure is often not enough, as the structure and properties of a material often change during device operation. Thus, it is necessary to understand how materials transform and degrade in response to operating conditions. This is especially true in materials for electrochemical energy devices, such as batteries, fuel cells, photocatalytic systems, or electrolyzers; in these devices, mass transport, high temperatures, and reactive environments are the norm and can accelerate material transformations. My group’s work is focused on developing and using in situ experimental techniques to investigate real-time material transformations down to the atomic scale, and determining how such changes influence device performance and lifetime. In this way, we are “peering inside” these energy devices to visualize materials behavior, which is a new way to understand energy materials.
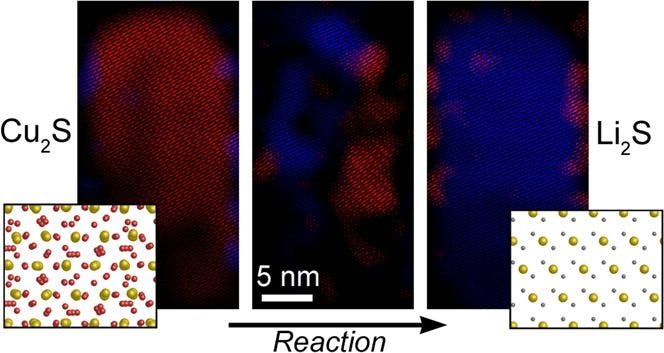
The dynamic processes we observe in materials include phase transformations, corrosion, nucleation and growth, and interdiffusion. These processes occur across different length and time scales and involve a wide variety of physical and chemical phenomena. Thus, multiple experimental tools are necessary to reveal overall behavior. My group’s go-to technique for investigating atomic-to-nanoscale processes is in situ transmission electron microscopy (TEM), and we are currently using this method to study transformations in materials for lithium-ion and sodium-ion batteries (Figure 1). TEM has been a powerful imaging technique in materials science for a long time, but traditional limitations (such as requiring a vacuum environment) largely prevented its use for studying transformations in materials. However, recent exciting developments have enabled imaging of materials exposed to liquids, gasses, and other electrochemistry-relevant environments, which has opened the door to investigating nanoscale dynamics during electrochemical reactions of materials. My group also uses other in situ methods that provide complementary information to TEM, including in situ x-ray diffraction for determining the structure and in situ x-ray photoelectron spectroscopy to probe solid-state chemical transformations. When combined with materials synthesis and conventional materials characterization methods, these techniques give us the power to gain a comprehensive understanding of the behavior of a variety of important materials under realistic conditions, which was impossible just a few years ago.
We are using these techniques to reveal operational and degradation mechanisms in materials for novel high-energy-density battery systems, devices for the electrochemical production of fuels, and new low-power ionic memory systems. Our results will let us understand how new materials in energy devices fail, and this will provide important guidelines for engineering longer-lasting, high-performance materials. We hope that the advances that we make in the lab will contribute to the development of new renewable energy technologies.