Professor Vern Schramm will present a talk and receive his award during the 256th ACS National Meeting & Exposition. The Gordon Hammes Award Lecture will take place on Sunday, August 19 beginning at 4:30 P.M. in Room 153B of the Boston Convention & Exhibition Center. The Award Reception will follow immediately after the Lectures in the same […]
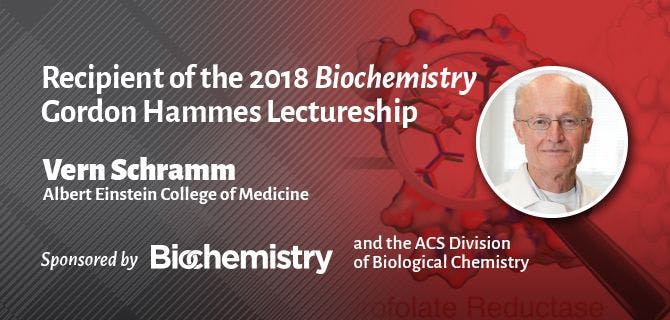
The Gordon Hammes Lecture Award is sponsored jointly by Biochemistry and the ACS Division of Biological Chemistry. The objective is to recognize and honor a single individual whose scientific contributions have had a significant impact on research at the interface of chemistry and biology. This year’s award goes to Professor Vern Schramm of the Albert Einstein College of Medicine.
“I am so incredibly thrilled that Vern has been selected as the winner of the Biochemistry 2018 Gordon Hammes Lectureship! The selection committee was unanimous and unanimously enthusiastic about his profound contributions to the field of biological chemistry”, says Alanna Schepartz, Editor-in-Chief of Biochemistry.
Schramm studied chemistry and microbiology for his B.S. at South Dakota State University. A scholarship for a master’s degree at the Harvard School of Public Health exposed him to nutrition and biochemistry. For his Ph.D. degree, he studied enzymatic mechanisms at the Australian National University with John Morrison during the time Morrison was deriving equations for tight-binding enzyme inhibitors.
Following an NRC-NSF postdoctoral fellowship at NASA Ames Research Center in California, Schramm began research in enzymatic reaction mechanisms and transition state analysis at the Department of Biochemistry, Temple University School of Medicine. In 1987 he was recruited to chair the Department of Biochemistry at the Albert Einstein College of Medicine, a position he held until 2015. He leads a research program in enzymology as Professor and Ruth Merns Chair of Biochemistry, the Schramm laboratory, which pioneered an approach to use intrinsic kinetic isotope effects combined with computational chemistry to understand the details of enzymatic transition states. Together with a team of chemistry collaborators in New Zealand, the Schramm laboratory designed and characterized the transition state analogs for a family of N-ribosyltransferases. These have proven to be powerful inhibitors. Three of the inhibitors designed by this approach have entered clinical trials, and others are in earlier development. Japan recently approved one analog for use in relapsed or resistant peripheral T cell lymphoma in Japan.
Read a Brief Interview with Gordon Hammes Lecture Award Winner Vern Schramm
How did you choose to pursue this field of research?
Ph.D. and postdoctoral training in enzyme kinetics taught the order and rates of steps enzymes take in their catalytic cycles. Excellent training, and still relevant to broad scopes of biomedical science. But it did not satisfy my curiosity about the details of the chemical step, the atomic nature of the transition state as it is formed in enzymatic catalytic sites. Then, reports in the chemistry literature indicated that the nature of transition states could be approached by combining kinetic isotope effects (how a heavy atom in the substrate changes the rate of chemistry) with quantum chemistry. Finally, we were able to solve enzymatic transition states. The level of detail we have now has almost satisfied my curiosity. Our lab is collaborative, and we rely on an excellent quantum chemist, Steve Schwartz at the University of Arizona for newly developing insights into fast atomic motions forming the enzymatic transition states.
What are you working on now?
Transition state theory teaches that knowledge of the transition states guides the design of chemically stable mimics of the enzymatic transition states. These are, in theory, the most powerful non-covalent inhibitors that can be designed to bind at catalytic sites. Many enzymes are drug targets. Selecting an enzyme that might be a suitable drug target, solving the transition state structure, designing powerful inhibitors and testing the inhibitors toward human clinical trials has become a growing focus. The chemical synthesis of transition state mimics is novel and difficult chemistry. A long-standing collaboration with chemists Peter Tyler and Gary Evans at the Ferrier Research Institute, Victoria University of Wellington, New Zealand is an essential part of this research work.
What do you anticipate working on in the future?
Cancer develops from a single aberrant cell that expands, accumulates new mutations, and thrives by altering gene expression (epigenetic) patterns to suit the ever-changing environment. Altering gene expression requires enzymatic alterations in nucleic acids and regulatory proteins. We are solving the transition states of epigenetic enzymes and searching for inhibitors as potential anticancer agents. An additional interest in probing deeper into the very fast atomic dance between atoms of the enzyme and the substrates to form the transition state.
What is important in training the next generation of researchers?
Generating curiosity for how and why things function as they do. Applying novel scientific approaches to solve unexpected questions as the research leads the investigator.
Explore some recent research articles from Vern Schramm
Promoting Vibrations and the Function of Enzymes. Emerging Theoretical and Experimental Convergence
Biochemistry, Article ASAP
DOI: 10.1021/acs.biochem.8b00201
Kinetic Isotope Effects and Transition State Structure for Hypoxanthine-Guanine-Xanthine Phosphoribosyltransferase from Plasmodium falciparum
Biochemistry, 2017, 56 (48), pp 6368–6376
DOI: 10.1021/acs.biochem.7b01027
Transition State Analogue Inhibitors of 5′-Deoxyadenosine/5′-Methylthioadenosine Nucleosidase from Mycobacterium tuberculosis
Biochemistry, 2017, 56 (38), pp 5090–5098
DOI: 10.1021/acs.biochem.7b00576
Hydride Transfer in DHFR by Transition Path Sampling, Kinetic Isotope Effects, and Heavy Enzyme Studies
Biochemistry, 2016, 55 (1), pp 157–166
DOI: 10.1021/acs.biochem.5b01241
Soapwort Saporin L3 Expression in Yeast, Mutagenesis, and RNA Substrate Specificity
Biochemistry, 2015, 54 (29), pp 4565–4574
DOI: 10.1021/acs.biochem.5b00405
Active Site and Remote Contributions to Catalysis in Methylthioadenosine Nucleosidases
Biochemistry, 2015, 54 (15), pp 2520–2529
DOI: 10.1021/bi501487w
Catalytic Site Cooperativity in Dimeric Methylthioadenosine Nucleosidase
Biochemistry, 2014, 53 (9), pp 1527–1535
DOI: 10.1021/bi401589n