Artificial dialysis mimics the kidneys’ function of filtering wastes from blood, which is crucial to keep patients who are suffering from advanced renal disease alive. Dialysis machines typically work by causing the body’s excess water, urea, creatinine, and other wastes to diffuse into a buffer solution, a process that requires approximately 6 L of dialysis […]
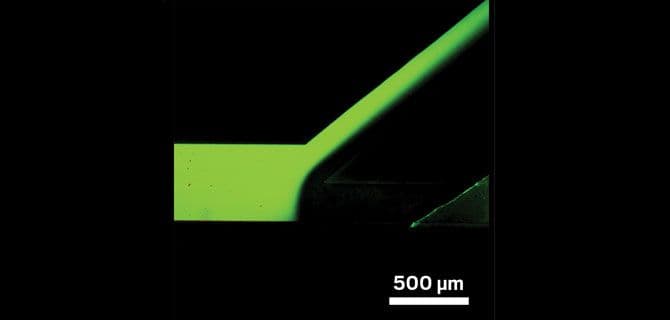
Artificial dialysis mimics the kidneys’ function of filtering wastes from blood, which is crucial to keep patients who are suffering from advanced renal disease alive. Dialysis machines typically work by causing the body’s excess water, urea, creatinine, and other wastes to diffuse into a buffer solution, a process that requires approximately 6 L of dialysis buffer for the procedure, which is required daily for many with kidney disease. Miniaturizing this reservoir has been the biggest barrier to creating a wearable artificial kidney. Now, researchers report that a new technique might be able to solve this problem much like the human kidney itself does: by drawing water directly from blood to continually replenish the dialysate buffer.
The method relies on a microfluidic device that uses electricity to separate charged and neutral molecules in circulating blood, explains chemist Robbyn K. Anand of Iowa State University. Anand developed the method during her graduate studies when working on seawater desalination. At the time, her brother suffered end-stage renal disease, inspiring her to adapt the method to dialysis for kidney disease patients.
During hemodialysis, the blood to be purified is typically separated from a reservoir of buffer by a semipermeable membrane. Waste products and excess water diffuse across this barrier until the buffer is saturated.
To reduce the volume of water needed, which could allow people who need dialysis to carry a continually operating system with them, people have tried to generate new buffer from the saturated buffer, not from blood, says nephrologist Jonathan Himmelfarb of the University of Washington, who was not involved with the study. This is an “innovative and different” approach.
Anand and her colleagues built a Y-shaped microfluidic device and placed a Nafion membrane, which is selectively permeable to cations, across one branch. When they applied a voltage across the device and allowed a liquid to flow toward the fork of the Y, cations flowed across the membrane and into an auxiliary channel. The arrangement creates a charge-depleted zone that deflects blood proteins and anions down the device’s other branch, concentrating these blood components. Water and other small molecules that can cross the membrane would be collected from the first branch to generate dialysis buffer.
The team tested the system using blood plasma containing the protein albumin linked to a fluorescent dye, and the dye-linked protein traveled into the channel away from the membrane, as expected. “We were able to identify voltage and flow conditions where 99% of proteins were excluded from the water,” Anand says. “That’s the most important feature that we need for this to be clinically relevant because protein loss is already such a problem for patients with kidney disease.”
In future work, the team aims to identify the molecules in the water that is extracted from blood. Before being put to clinical tests, the method will also need to be scaled up: At present, the microfluidic device only processes a few hundred microliters of blood at a time. The team is also working to identify biocompatible alternatives to the Nafion membrane, which can’t be used in medical devices. Nonetheless, the results suggest that in the future, it might be feasible to extract water from blood to create a dialysis buffer. “This is a proof of concept worth exploring,” Himmelfarb says.