Discover how increasing wildfire severity is driving the use of fire suppression products, potentially leading to significant environmental contamination from toxic metals.
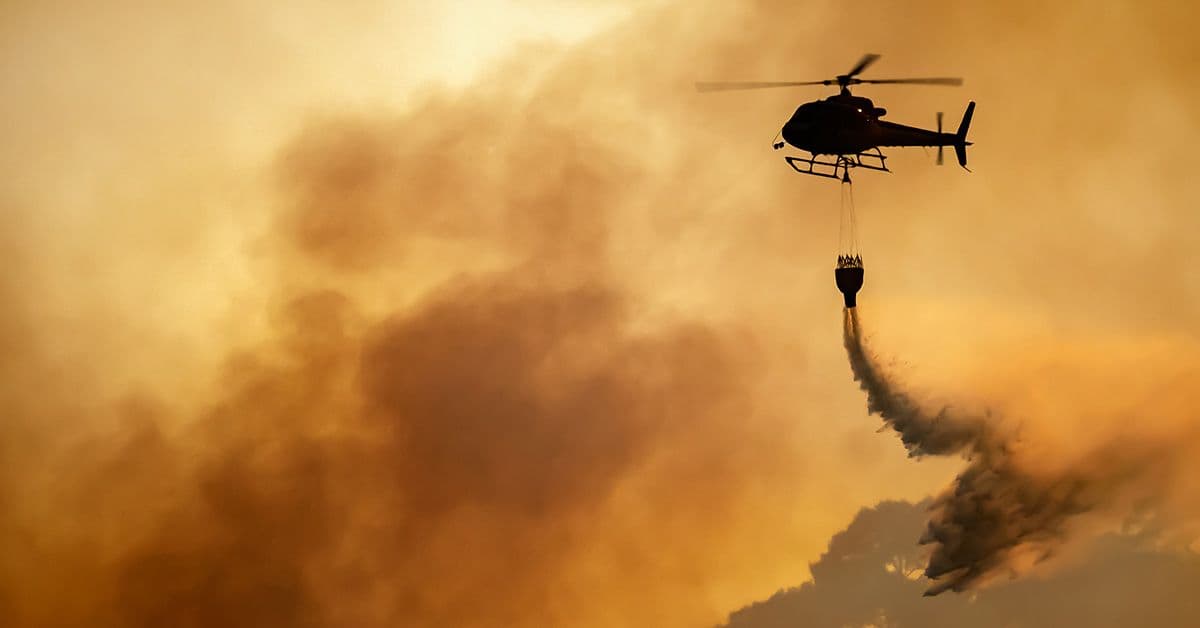
Wildfires are increasing in frequency and severity, with a 50% increase in seasonal wildfire severity predicted in the United States in the coming decades.1 Globally, 3.9×106 square kilometers of land burned in the 2023–2024 season, generating fire carbon emissions 16% above average.2 By the end of the century, it is estimated that events of similar magnitude to the Canadian wildfires seen in 2023 will occur somewhere between 6 and 11 times more frequently under a medium–high emission scenario.2 Rising wildfires have led to increased application of fire suppression products in an effort to contain and minimize damage—but at what cost?
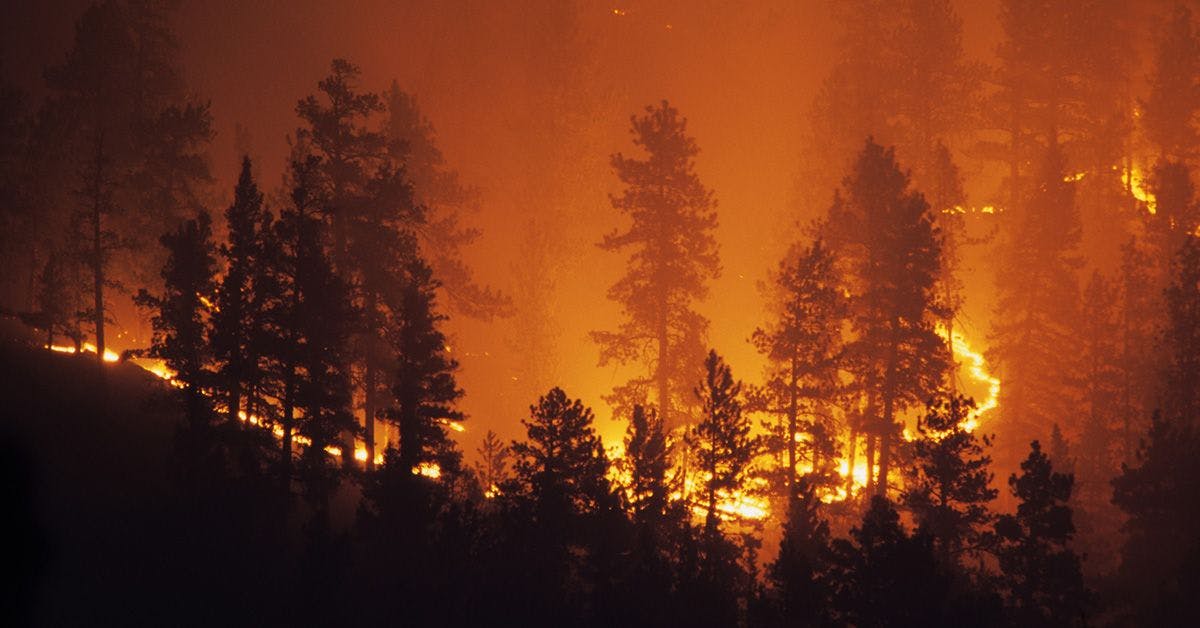
Understanding the Health and Environmental Impacts of Wildfires
In the United States, products used on wildfires must be approved by the U.S. Forest Service—but some formulations are trade secrets, so it is not always possible to know what is being applied to the land in long-term fire retardants, water enhancers, or Class A foams. However, we do know that the active ingredients in long-term fire retardants are typically salts—often fertilizers such as ammonium polyphosphate—which react with cellulose to form a protective char layer.3-5 This char slows fire spread and decreases intensity, even after the water in the retardant evaporates. Increased metal content in soils and surface water has been observed after wildfires, but has often been attributed to ash or anthropogenic impact rather than the chemicals used to fight the fires. For example, at one monitoring station 200 km away from a wildfire, lead levels were 40 times higher than average, but this was thought to be due to the combustion of houses and vehicles.6
Despite the absence of published data on metals in fire suppressants, two pieces of circumstantial evidence highlight them as potential sources of environmental contamination. In 2016, an air tanker base in Washington State where fire suppressants were stored was cited for multiple waste discharge permit violations, including exceeding allowable metal concentrations.7 Additionally, an internal U.S. Bureau of Land Management guidance document for tanker bases cautions that “retardant contains ammonia, cadmium, and chromium.”8
To unpick this and gain greater clarity, a team from California have quantified the metal concentrations in several fire suppression products to evaluate whether these could be contributing to increased metal concentrations in the environment.9 The results showed that long-term fire retardants contained concentrations of toxic metals up to 2,880 times greater than drinking water regulatory limits, and potentially greater than some aquatic toxicity thresholds when released into the environment. This included antimony, arsenic, barium, cadmium, chromium, copper, lead, manganese, thalium, and vanadium. Water enhancers and Class A foams also contained some metals, but at lower concentrations than fire retardants. Based on these concentrations and retardant application records, the authors estimate that use of fire retardants in the U.S. alone released around 380,000 kg of toxic metals into the environment between 2009 and 2021.
Wildfires can also lead to substantial loss of mercury that was previously sequestered in vegetation, surficial detritus, and topsoil, releasing it unto the atmosphere predominantly as gaseous elemental mercury. A 2018 article on the origin, reactivity, and bioavailability of mercury from wildfires also notes that ash is highly susceptible to leaching,10 suggesting a strong potential for transporting metals—regardless of their origin—to aquatic environments including streams, lakes, and reservoirs.
Crucially, the authors of the current work also hypothesize that metals are being used in these fire products as corrosion control agents. So is there an alternative? We recently looked at how biowastes such as lignin in cocoa pod husks could be used as an eco-friendly fire retardant. Perhaps with greater clarity on what is being used and why, we can identify more effective and less harmful solutions.
Further Explorations: Wildland Fires
To shine a spotlight on current trends within this crucial topic area, ACS Publications is pleased to share two curated collections of cutting-edge developments published across our Environmental Science journal portfolio.
A recently published Special Issue, "Wildland Fires: Emissions, Chemistry, Contamination, Climate, and Human Health," highlights the latest discoveries on the environmental and health impacts of wildland fires. This ongoing rolling collection will continue to update as additional papers complete the peer review process, and we encourage you to periodically revisit the Special Issue page for the latest studies.
Another retrospective Collection, "Emissions, Chemistry, and the Environmental Impacts of Wildland Fire," includes articles from 2019-2024 published in ACS ES&T Air, ACS ES&T Water, Environmental Science & Technology, and Environmental Science & Technology Letters. This Collection showcases the breadth and complexity of wildland fire research,11 covering wildland fire emissions, impacts on the wildland/urban interface, brown carbon production, the atmospheric evolution of wildland fire smoke, source apportionment studies, impacts on human health and ecosystems, and advances in smoke modeling.
References
- Flannigan, M.D. et al. Climate Change and Forest Fires. Sci. Total Environ. 2000, 262, 221– 229.
- Jones, M.W. et al. State of Wildfires 2023–2024. Earth Syst. Sci. Data 2024, 16, 3601–3685.
- Ecological Risk Assessment of Wildland Fire-Fighting Chemicals: Long-Term Fire Retardants. Prepared for Fire and Aviation Management & National Technology and Development Program, U.S. Forest Service: Denver, CO, November 2021.
- Speight, J.G. Chapter 4 - Sources and Types of Organic Pollutants. Environmental Organic Chemistry for Engineers. Butterworth-Heinemann, 2017, 153-201.
- Camino, G. et al. Overview of Fire Retardant Mechanisms. Polym. Degrad. Stb. 1991, 33, 131– 154.
- Boaggio, K. et al. Beyond Particulate Matter Mass: Heightened Levels of Lead and Other Pollutants Associated with Destructive Fire Events in California. Environ. Sci. Technol. 2022, 56, 20, 14272–14283.
- Moses Lake Air Tanker Base Regional Engineering Report. U.S. Forest Service, Moses Lake, WA, 2016.
- Mobile Retardant Base Stormwater Pollution Prevention Plan. U.S. Bureau of Land Management: Denver, CO, 2021.
- Schammel, M.H. et al. Metals in Wildfire Suppressants. Environ. Sci. Technol. Lett. 2024, 11, 11, 1247–1253.
- Ku, P. et al. Origin, Reactivity, and Bioavailability of Mercury in Wildfire Ash. Environ. Sci. Technol. 2018, 52, 24, 14149–14157.
- Holder, A.L. and Sullivan, A.P. Emissions, Chemistry, and the Environmental Impacts of Wildland Fire. ACS EST Air 2024, 1, 9, 946–950.