Solar technology has traditionally relied on large, heavy panels that are costly and have a limited lifespan. But the science has quietly been evolving to more efficient, lightweight materials—and now, perhaps, from outside our homes to in.
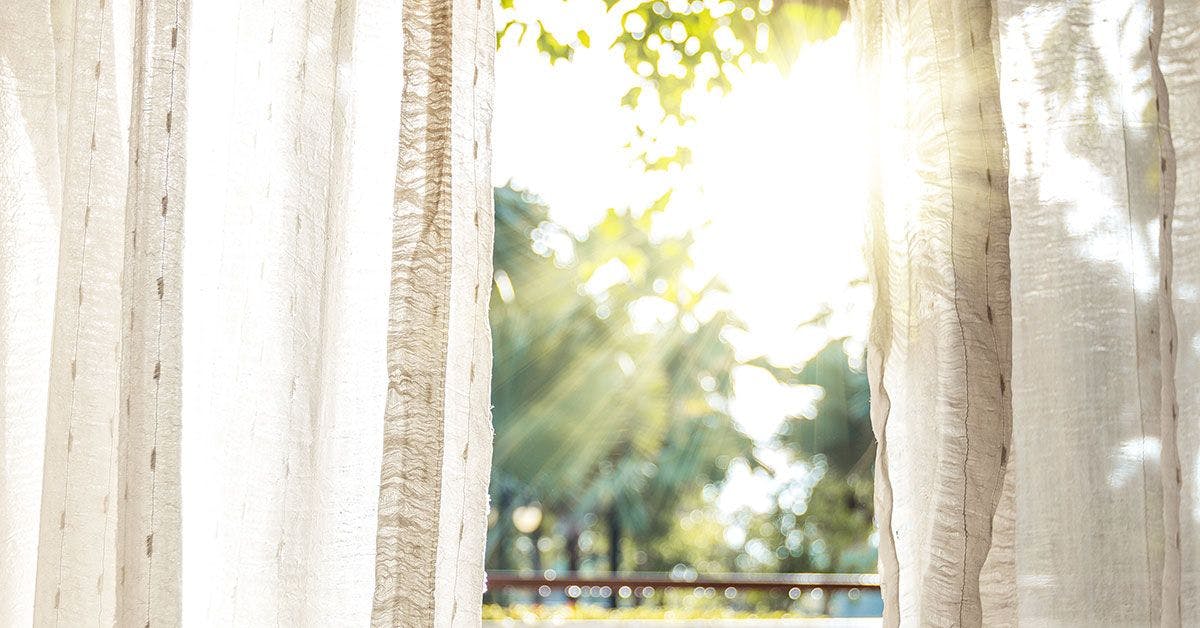
If you think of solar power, you likely imagine large, gridded panels on rooftops or in neat dark rows across farmland, quietly harvesting energy from the sun. But this technology may soon begin making its move indoors as a source of power for our growing army of digital devices. That sounds revolutionary, but in fact we’ve been doing this for a while— if you have owned a basic, low-powered desk calculator any time since the late 1970s, it likely was running on solar cells embedded in the front panel. But many of our small electronics today rely on batteries, with associated issues of resource use and disposal. Indoor photovoltaics make sense—but can they make enough energy to power the "Internet of Things"?
There are key differences between natural sunlight and artificial indoor light. One drawback of sunlight is that it depends on weather, latitude, altitude, season, or time of the day, whereas indoor light is stable – although varies depending on lightbulb type such as fluorescent, halogen, or LED. Solar irradiance is also typically many orders of magnitude more intense than indoor illumination, and is also across a much wider spectrum. However, even very small amounts of indoor light could be enough to power a large number of our internet-of-things devices, which often have very low power consumption.1
In a recent issue of ACS Applied Energy Materials, David Müller and colleagues published a comparison of state-of-the-art photovoltaic technologies.2 Previous work by members of the team has looked at photogenerated and electrode-induced charges in optimized organic solar cells,3 as well as low-temperature routes.4
In this latest study, the researchers compared several different photovoltaic technologies under identical indoor LED illumination. The materials included amorphous and crystalline silicon, copper indium gallium selenide, cadmium telluride, III–V, organic, dye-sensitized, and perovskite.
Crucially, when looking at indoor photovoltaics, there is a need for different measures to those suitable for outdoor panels; whereas optimal band gaps of 1.15–1.34 eV are appropriate for sunlight, this shifts to 1.8–2.0 eV for indoor cells. Results of the comparison showed that absorber materials with larger band gaps had fewer thermalization losses, and could reach higher power conversion efficiencies. This is important, since thermalization and non-absorption are the two most fundamental loss mechanisms for solar cells. The best device tested was a gallium indium phosphide cell, which achieved record efficiency of 39.9% under 500 lx cold white LED light.
Similar results have previously been reported for transparent hydrogenated amorphous silicon solar cells, with maximum efficiency of 36%. In that study, the cells also showed excellent long-term performance, sustaining over 99% of original efficiency under continuous indoor light illumination for 200 hours.5
Considerations remain. Fabrication processes will need to find the optimal thickness, since for some materials—including dye-sensitized cells—this can affect diffusivity, ionic conductivity, and charge transfer resistance at the electrolyte interface.6 A further consideration is UV stability, since prolonged light exposure can cause burn-in—resulting in a reduction in photocurrent at high photon energies.7 However, it looks like dye-sensitized perovskite or organic solar cells will become the preferred candidates to provide cheap photovoltaics for low-power internet-of-things devices.2,8 In fact, it has estimated that the perovskite solar technology will make up more than 29% of the global photovoltaic market by 2030.6
Another article published in ACS Energy Letters discusses the applications of indoor photovoltaics based on perovskite, suggesting a role in the home for automation and security devices, as well as in powering sensors to detect temperature and humidity.8 It is predicted we will see them incorporated into our portable lifestyle electronics—removing the need to plug in and charge our smart watches or earphones, or to replace the batteries in the wireless mouse or smoke alarm.
References
- Motlagh, H. N. et al. Internet of Things (IoT) and the Energy Sector. Energies. 2020, 13 (2), 494.
- Müller, D. et al. Indoor Photovoltaics for the Internet-of-Things – A Comparison of State-of-the-Art Devices from Different Photovoltaic Technologies. ACS Appl. Energy Mater. 2023, 6, 20, 10404–10414.
- Würfel, U. et al. Recombination between Photogenerated and Electrode-Induced Charges Dominates the Fill Factor Losses in Optimized Organic Solar Cells. J. Phys. Chem. Lett. 2019, 10, 12, 3473–3480.
- Schulze, P. C. S. et al. Novel Low-Temperature Process for Perovskite Solar Cells with a Mesoporous TiO2 Scaffold. ACS Appl. Mater. Interfaces 2017, 9, 36, 30567–30574.
- Kim, G. et al. Transparent Thin-Film Silicon Solar Cells for Indoor Light Harvesting with Conversion Efficiencies of 36% without Photodegradation. ACS Appl. Mater. Interfaces 2020, 12, 24, 27122–27130.
- Venkatesan, S. et al. Indoor Dye-Sensitized Solar Cells with Efficiencies Surpassing 26% Using Polymeric Counter Electrodes. ACS Sustainable Chem. Eng. 2022, 10, 7, 2473–2483.
- Patel, J. B. et al. Effect of Ultraviolet Radiation on Organic Photovoltaic Materials and Devices. ACS Appl. Mater. Interfaces 2019, 11, 24, 21543–21551.
- Wojciechowski, K. and Forgács, D. Commercial Applications of Indoor Photovoltaics Based on Flexible Perovskite Solar Cells. ACS Energy Lett. 2022, 7, 10, 3729–3733.