Society is moving toward smart electronics that require flexibility, resilience, and safety—and a sustainable and cost-effective way to keep them running. Several recent studies have demonstrated the potential for sodium-ion batteries to one day be a practical, cheaper replacement for current lithium-ion technology.
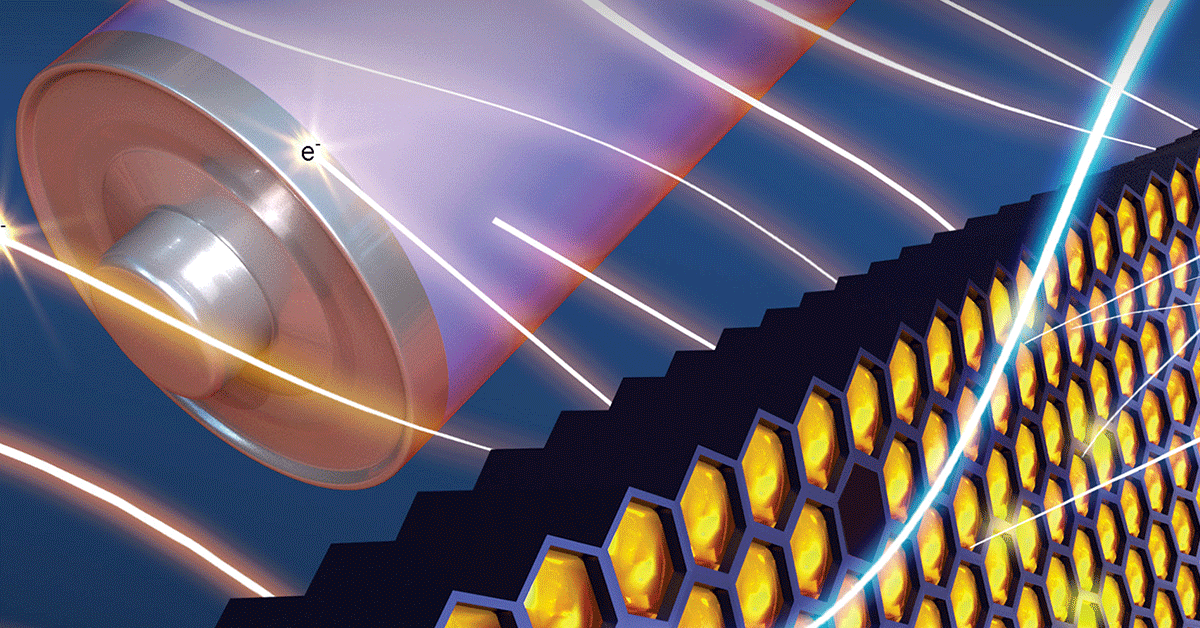
The Lithium Problem
Batteries are everywhere. From toothbrushes to cars, batteries are responsible for powering many of the devices that help us go about our daily lives without constantly needing to be plugged in to an electrical outlet. If you are currently using a laptop or portable device, there’s a likely chance you are reading this post thanks to battery power.
Over the past several decades, lithium-ion batteries (LIBs) have come to dominate the rechargeable battery industry and are now the go-to power source for the majority of portable electronic devices and gadgets. LIBs far outperform other rechargeable alternatives due to their high energy density, ability to hold a charge over long periods of time, and resilience to frequent charge cycles.1
As beneficial as they are, lithium-ion batteries also come with significant drawbacks that may hinder long-term production and applications. The limited availability of lithium, cobalt, and other raw materials cannot meet the growing global demand for lithium-ion technology—and the extraction process is costly, hazardous, and environmentally detrimental. Furthermore, despite LIBs being generally safe, they are still susceptible to overheating—or in some cases, exploding—if exposed to certain conditions.
But now, new studies aim to identify viable lithium-free battery alternatives in hopes of establishing cheaper, safer, and more environmentally sustainable options for powering our devices in the future—and a number of researchers have developed a particular interest in sodium-ion technology as a potential replacement.2
Advantages and Challenges of Sodium-Ion Technology
Sodium-ion batteries (SIBs) offer several key advantages compared to their lithium-ion counterparts. In terms of raw material availability, sodium is the clear winner. It is at least one thousand times more abundant in the earth’s crust than lithium and much cheaper to obtain.3 It can also be extracted through more environmentally friendly processes such as seawater desalination.
Despite these advantages, there are still significant hurdles in the way of more widespread, practical SIB applications. One challenge involves the limitations of sodium-based layered transition metal oxides (TMOs) as cathode materials—notably, lower specific capacity and energy density resulting from a more convoluted charge-discharge cycle.4
One recent study explores anionic redox reaction (ARR) as a favorable method to boost capacity and improve the overall performance of SIB cathode materials. Although ARR-based cathode materials are encouraging for greater storage capacity, other challenges still remain, and more research is necessary to fully understand the fundamental chemistry of ARR before it can be successfully applied within SIB technology.4
In addition to lower specific capacity and energy density compared to LIBs, liquid SIBs are susceptible to an array of safety hazards that have severely hindered future developments and progress toward commercialization. Researchers have proposed solid-state sodium metal batteries (SSMBs) as suitable alternatives due to their improved energy density, lower cost, and high stability. However, their solid-state electrolyte (SSE) components often demonstrate low ionic conductivity and various interfacial compatibility challenges, which can ultimately impede electrochemical performance.5
With these challenges in mind, sodium- or zinc-based hybrid supercapacitators may become an appealing option for commercial applications by providing a mashup of two different energy storage technologies. By combining a capacitator-type cathode with a battery-type anode, these hybrid storage devices can achieve high energy density without compromising stability or power delivery—but challenges remain even here, and more research is crucial for making pragmatic hybrid supercapacitator applications a reality.6
A Better Way to Power Our Lives
Although sodium remains a top contender for next-generation batteries, the technology is not quite there yet. Identifying more effective cathode materials will help to build powerful, stable SIBs with greater specific capacities. Further research should also focus on advancing battery electrolyte technology so that SIBs can successfully perform over a wide temperature range while maintaining the long charge cycles and lifespans required for large-scale energy storage.3
As we continue progressing toward widespread sustainable energy sources such as wind or solar power—all of which depend on battery storage for uninterrupted performance—researchers are optimistic that sodium-ion technology will pave the way toward more cost-effective, sustainable, and powerful batteries.
Explore More Articles on Sodium-Ion Batteries in ACS Journals
- Gupta, P. et al. Understanding the Design of Cathode Materials for Na-Ion Batteries. ACS Omega 2022, 7, 7, 5605–5614.
- Hakim, C. et al. Anionic Redox and Electrochemical Kinetics of the Na2Mn3O7Cathode Material for Sodium-Ion Batteries. Energy Fuels 2022, 36, 7, 4015–4025.
- Li, Q. et al. Reforming Magnet Waste to Prussian Blue for Sustainable Sodium-Ion Batteries. ACS Appl. Mater. Interfaces 2022, 14, 42, 47747–47757.
- Mishra, R. Single-Source-Derived Nitrogen-Doped Soft Carbons for Application as Anode for Sodium-Ion Storage. Energy Fuels 2022, 36, 12, 6483–6491.
- Thangavel, R. et al. Emerging Materials for Sodium-Ion Hybrid Capacitors: A Brief Review. ACS Appl. Energy Mater. 2021, 4, 12, 13376–13394.
References
- Manthiram, A. An Outlook on Lithium Ion Battery Technology. ACS Cent. Sci. 2017, 3, 10, 1063–1069.
- Ponnanda, S. et al. Lithium-Free Batteries: Needs and Challenges. Energy Fuels 2022, 36, 12, 6013–6026.
- Abraham, K. M. How Comparable Are Sodium-Ion Batteries to Lithium-Ion Counterparts? ACS Energy Lett. 2020, 5, 11, 3544–3547.
- Wu, Q. et al. Anionic Redox Chemistry for Sodium-Ion Batteries: Mechanisms, Advances, and Challenges. Energy Fuels 2022, 36, 15, 8081–8095.
- Li, Z. et al. Solid-State Electrolytes for Sodium Metal Batteries. Energy Fuels 2021, 35, 11, 9063–9079.
- Shaikh, N.S. et al. Recent Advancements in Energy Storage Based on Sodium Ion and Zinc Ion Hybrid Supercapacitors. Energy Fuels 2021, 35, 18, 14241–14264.