Chemistry spans all disciplines, and and art is no exception. New research now paints a clearer picture of how the transition from egg tempera to oil paint in the 15th century—and the combination of both materials—impacts modern conservation techniques.
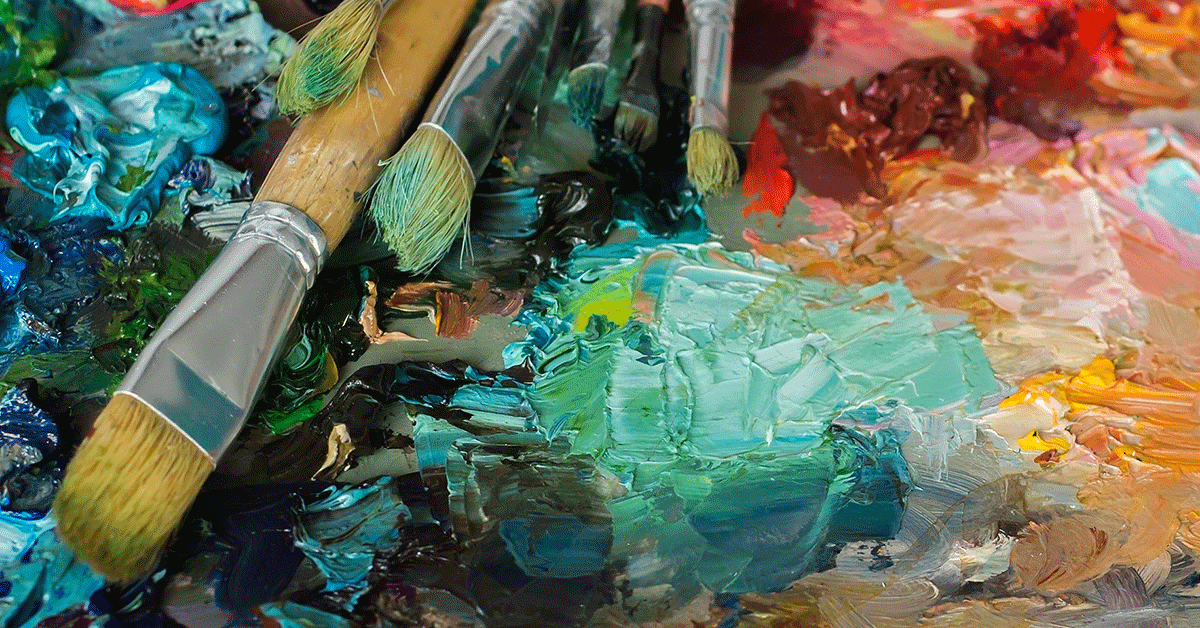
Paint consists of fine pigment particles dispersed in an organic fluid binder. In the 14th century, the binder was traditionally egg, but just 100 years later there was a move to oil-based paints such as linseed, which we might be more familiar with today. The key difference between egg-based (tempera) paints and oil paints on the canvas is that tempera paints dry very quickly due to water evaporation. In order to achieve color shifts and transitions, different colors of these tempera paints were applied on top of each other in many translucent layers or thin hatched lines. In contrast, oil paints cure slowly by chemical cross-linking, so transitions can be achieved by wet-in-wet blending of different colors into each other.
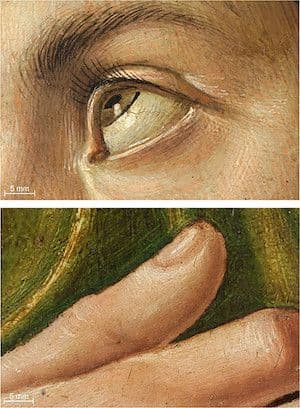
As with many technology shifts, there was a degree of crossover in the 15th century, with some artists using tempera and oil in different areas of the same paintings (as seen above) or mixing both together in a medium called fatty tempera, or tempera grassa. And now, researchers working in Italy and Germany are investigating the microstructure, rheology, drying kinetics, and chemistry of these different paint materials. While it has been the norm to define a painting as tempera grassa based only on visual examination, this new work could help art historians more thoroughly understand these transition works and provide a framework to rationalize the influence of paint composition and preparation on the finished work.
Findings show that, despite their hydrophobic nature, pigments do not enter the oil droplets emulsified in tempera grassa due to the egg proteins on their surface, with minor differences in viscosity attributed to the broad particle size distribution. The result is that tempera grassa paints remain liquid long enough to fuse and then level after application. Interestingly, the added oil does not change the general behavior or drying time, but does result in different brushability on absorbent surfaces. This is because the water in the paint is lost quickly, but the oil keeps it soft. Once tempera grassa is dry to the touch, oil cross-linking sets in, leading to a second hardening step. But this isn’t always the end of the story for the paint surface—and there have been reports of paintings dripping many years after being completed.
Other groups have also looked at the chemistry of this cross-linking process in oil paint, with the aim of understanding the molecular processes that convert the fluid binder into a dry film and how this evolves over time. This is also important, as the resulting film has a direct relationship with paint stability over the long term. Here, chemistry offers the ability to study the kinetics of the oil oxidation and estimate the oxidative degradation using isothermal thermogravimetric analysis, or to monitor the presence of hydroperoxides and active radicals with differential scanning calorimetry. Gas chromatography–mass spectrometry also has a role in investigating noncovalently cross-linked fractions. Findings suggest that, although a cross-linked network may form upon curing, some triglycerides fail to cross-link to the wider network and oxidize to form acidic and hydroxylated polar moieties. When these oxidized species cannot chemically bind with pigments, they are free to migrate to the paint surface—leading to exudates, drips, and tackiness.
These efforts to help unpick the chemistry of the Great Masters’ brushstrokes are crucial for art historians and custodians. Conservation challenges can be unpredictable, but understanding the original materials and artistic practice helps support restoration and preservation of artworks for future generations.
Watch the video on this research created by the ACS Science Communications team: