Molly Stevens is Professor of Biomedical Materials and Regenerative Medicine and the Research Director for Biomedical Material Sciences in the Department of Materials, Department of Bioengineering and the Institute of Biomedical Engineering at Imperial College London. In 2004, Professor Stevens was included in the TR100 list of the top innovators under 35 who are transforming […]
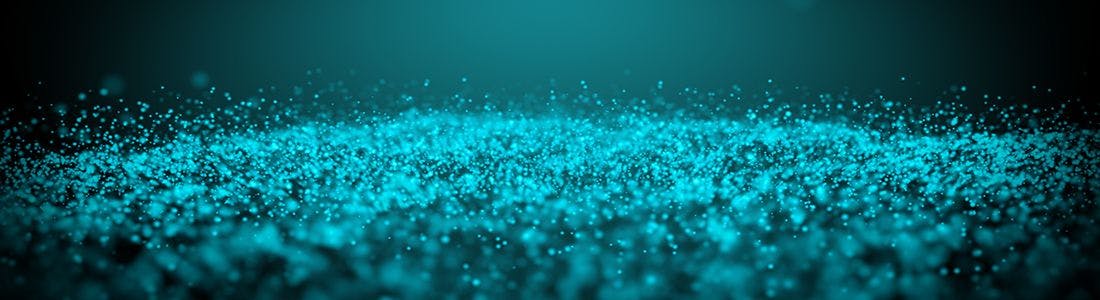
In 2004, Professor Stevens was included in the TR100 list of the top innovators under 35 who are transforming technology — and the world — with their work. In 2010, The Times of London named her one of the top 10 scientists under 40. She also received the Polymer International-IUPAC award for creativity in polymer science, the Rosenhain Medal, and the Norman Heatley Prize for Interdisciplinary Research from the Royal Society of Chemistry. Her interdisciplinary work is highly cited, and she is included in Clarivate Analytics Highly Cited Researchers list in the cross-field category. In 2016, Stevens was recognized by the Society for Biomaterials with the Clemson Award for Basic Research, and she received the Rosalind Franklin Medal from the Institute of Physics in 2018.
Professor Stevens is an Associate Editor of ACS Nano and has served on the Board of Reviewing Editors of Science. She is a Fellow of 7 U.K. Societies, including the Royal Society of Chemistry and the Royal Academy of Engineering. In 2019, she was elected a Foreign Member of the National Academy of Engineering (USA). In 2020, she was elected Fellow of The Royal Society.
The Stevens Group is a large and extremely multidisciplinary research group of students, postdocs, and research fellows. They use innovative bioengineering approaches to pursue their vision of solving key problems in regenerative medicine and biosensing. Their research spans drug delivery, bioactive materials, tissue engineering, biosensing, materials characterization, soft robotics, and the interface between living and non-living matter, and is underpinned by collaborations with data scientists and molecular dynamics experts. This work is inherently interdisciplinary, so the group is made up of a diverse cast of materials scientists, engineers, chemists, biologists, physicists, and surgeons. The group’s work has a host of applications across diseases ranging from cancer to global health applications.
In this interview, I talk to Professor Molly Stevens about her early career, how she came to focus on regenerative medicine, and the importance of democratizing access to healthcare technologies.
What are some discoveries early in your career that has led to your current research in regenerative medicine?
During my Ph.D. I worked on very fundamental biophysics research about single molecules. We were some of the first to measure the forces which hold small proteins together. Although these types of scientific measurements are important for our understanding of biology, for my postdoc, I wanted to take a step towards some real-life applications and tackle important research challenges in developing materials that can heal the body. I did this work in Professor Bob Langer’s lab at MIT and afterward started my own research group at Imperial College London.
My research interests have widened from biomaterials into a plethora of new bioengineering solutions to help heal the body and detect diseases and work on innovative ways to characterize and study biomaterials. Members of my team still work on my initial area of interest – the fundamental biophysical mechanisms – so now we enjoy the best of both worlds; fundamental research across length scales and bioengineering approaches to regenerative medicine at the human scale. I think the symbiosis of fundamental research and engineering applications is very motivating and super exciting.
Can you please tell me more about your work with stem cells? What were your initial thoughts when you began using them in your research?
Some of my earliest research centered around stem cells and their use in tissue engineering. Tissue engineering – or engineering materials to encourage new biological tissue growth – is traditionally carried out in the laboratory. The patient’s stem cells are grown artificially into tissue in the laboratory and then implanted where needed. But, together with collaborators in the Langer lab, we thought, ‘why not use the body’s own capacity for growth and regeneration to make a laboratory inside the body itself?’ We proved that concept using bone tissue and have been innovating with stem cells ever since.
Fast forward to today, and we have engineered materials to guide stem cells for bone, cardiac tissue, cartilage, and even neural tissue, to not only differentiate into the cells of these tissues but also engineer their tissue structure, creating gradients and textures which mimic those found in the body. We are working on translating this research into the clinical setting and changing the lives of patients.
When I started working with stem cells, I was just fascinated by the complexity of even a single cell. We still have so much to discover about how cells can interact with engineered materials – this field still holds so much potential.
Can you please tell us about peptide-functionalized nanoparticles for enzyme biosensing? What are some of its applications that the general public may be familiar with?
Nanoparticles are roughly a million times smaller than a full-stop. We use small gold clusters as biosensing systems, which are programmed with peptides to detect diseases such as cancer and HIV in the body. It works like this; gold clusters are functionalized with peptides to create a relatively large ‘complex’, and these are injected into the body. In healthy people, these complexes are too large to be broken down by the kidneys and pass through into the urine. Diseases such as cancer and HIV produce distinct enzymes, whose job is to break down proteins. By matching the proteins on the complex with those targeted by disease enzymes, complexes injected into diseased patients get broken down by the enzymes into smaller components. Once this happens, the gold clusters are then small enough to pass through the kidneys and into the urine. A simple test can produce a color change reaction of the urine to turn it blue in patients with the targeted disease markers. This work is in the pre-clinical research stage in collaboration with the Bhatia group at MIT.
Interestingly, gold nanoparticles aren’t new. They have been used since Antiquity for their abilities to generate interesting colors dependent on their size; the Romans used gold nanoparticles to make color-changing glass, for example, in the Lycurgus Cup, which can now be seen in The British Museum. Medicinal use of this so-called ‘colloidal gold’ began in the Middle Ages, and we are using it in advanced diagnostics today. However, we have taken it one step further by adding platinum as a nanocatalyst to make the system many times more sensitive to disease. By applying these onto a paper-based lateral flow assay – similar to a home pregnancy test – we can produce cheap, reliable, and sensitive methods of detecting disease at the very early stages, with applications from cancer to infectious disease.
What is a problem that you solved during your most recent project? What does this solution mean for your research?
Many of the tissues and materials we study are at the nanoscale, and we are constantly pushing at the very limits of what current characterization technologies can achieve. For example, what happens when you want to study both the chemical and the physical characteristics of a single nanoparticle? Those would normally need different analysis techniques which would use different sample preparation; the data would often be based on bulk populations, not single particles. We’ve found that sometimes existing characterization technologies can’t give us the data we need, so we built our own instruments and developed our own analysis techniques.
One really exciting example of this is our SPARTA® technology – it stands for Single Particle Automated Raman Trapping Analysis. It’s an instrument that uses a laser to trap single nanoparticles, and that same laser can be used for Raman spectroscopy. Our single instrument can decode information such as chemical composition, particle size, and surface chemical reactions in real-time for individual particles. This system’s real power is that this can all be done in an automated way, with high throughput and the methods are non-destructive and label-free.
SPARTA® is really useful for our study of nanoparticles for drug delivery and nanodiagnostics. But we recognize that it could also be used for research in a broad range of fields, from gene therapy to the analysis of ocean nanoplastics. We’re currently working to translate our developmental research into a commercial product, which we anticipate will be taken up by all sorts of researchers in academia and industry. It’s exciting to think that our lab’s work could help further many other areas.
What do you think is the most interesting and/or important unsolved problem in your field?
I’m really keen to see the democratization of access to healthcare technologies. This is something that has motivated and driven me for a really long time. I’ve traveled and spent extended time in developing countries and seen the pressing need for and the potential impact that bioengineered technologies could have. In particular, I believe mobile smartphone technology is an enormous opportunity to help us empower people, connect them around the world, and help with early detection of disease.
Because our diagnostic tests’ outcomes are shown in visual color changes, we are working on using the camera from a smartphone to gather data at the population level. Our app is able to interpret the results from the diagnostic test and register the patient’s geo-location. These data can be used during and after epidemics of infectious diseases such as Ebola to effectively track, trace, and treat an outbreak. We’ve been working on this closely with teams in Uganda and South Africa.
Our diagnostic technology is only one piece of the puzzle; we also have exciting ongoing work to develop low-cost regenerative medicine implants with partners in a number of developing countries. It’s crucial for us to work with people locally who have the knowledge and resources to set up the infrastructure for this. Another challenge is the evolving regulations and how we handle patient privacy on these platforms. Now is a very exciting time because it’s all evolving in front of us.
Have you received any good advice that stuck with you? How has it helped you within your career?
I’ve been fortunate to have had so many mentors and supporters over my career. Their best advice has been to focus on really important problems as well as nurture a fantastic team.
What advice would you give people who want to pursue a career in science? If you had to start over again, what advice would you give yourself?
Finding an area of science that you really believe in is incredibly important, as well as building a brilliant team to help you get there. I am thankful every single day for how wonderful my team and collaborators are. If I was starting over, I would remind myself that every setback is just an opportunity to learn. This is what I tell my trainees, and I also truly believe it.