The recipients of 2022 The Journal of Physical Chemistry and PHYS Division Lectureship Awards are: Jonas Elm, Aarhus University, Denmark; Krupa Ramasesha, Sandia National Laboratories, USA; and Joel Yuen-Zhou, University of California San Diego, USA.
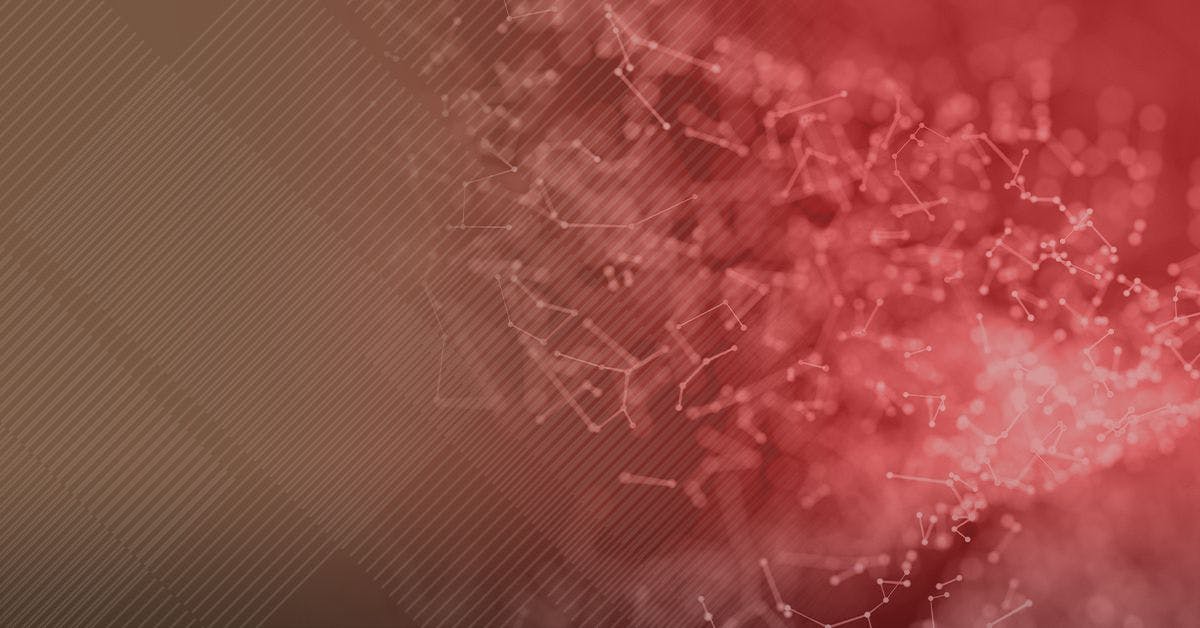
The recipients of 2022 The Journal of Physical Chemistry and PHYS Division Lectureship Awards are:
- Jonas Elm, Aarhus University, Denmark
- Krupa Ramasesha, Sandia National Laboratories, USA
- Joel Yuen-Zhou, University of California San Diego, USA
I spoke with the recipients to find out what the award means to them.
Jonas Elm – The Journal of Physical Chemistry A Recipient
Jonas Elm received a PhD degree in theoretical chemistry from the University of Copenhagen (2014) working with computational modelling of atmospheric molecular clusters under the supervision of Prof. Kurt V. Mikkelsen and Merete Bilde. In 2014 he joined as a postdoc in the center for exploitation of solar energy at the University of Copenhagen, where he computationally screened for new target photoswitch molecules for application as solar heat batteries. In 2015 he joined the group of Prof. Hanna Vehkamäki at the University of Helsinki, where he was working as a postdoc modelling atmospheric new particle formation using quantum chemical methods.
In 2017 he started a postdoc position at Aarhus University working with Prof. Merete Bilde modelling iodine reaction kinetics. In 2020 he was employed as assistant professor at Aarhus University to initiate an independent research group. The overarching goal of the research group is to implement more theoretically sound chemical schemes into atmospheric models. His research group is currently striving towards developing a unified atmospheric new particle formation model using a combination of quantum chemical calculations and machine learning methods.
What do you consider to be the most important advancement in physical chemistry in the past five years?
I consider the development and application of machine learning (ML) models to quantum chemical (QC) datasets as one of the most important advances in physical chemistry in the recent years. Modern ML models provide an important complimentary tool to expensive QC calculations. Hence, now it is possible to boost QC calculations with data-driven approaches far beyond what was possible five years ago. We are currently working on applying ML to study atmospheric cluster formation and initial results look extremely promising.
What advances has your lab made in the past five years?
In the recent years our research aim has been to study atmospheric new particle formation. Especially, the modelling of the initial formation of atmospheric molecular clusters has been our prime research. Atmospheric molecular clusters are difficult to model, as there are many combinations of molecules that might be relevant, and each of the combination can further exist in numerous configurations.
We recently outlined a holistic approach, coined clusteromics, which involves the comprehensive screening of small multi-component molecular clusters. Such an approach will simultaneously yield direct insight into the chemical species involved in cluster formation while assisting in the construction of thermochemical databases that can act as basis for quantum machine learning. Hence, in the recent years we have significantly advanced the field by improving the understanding of which chemical species that are involved in the initial cluster formation steps and paved the way for more data-driven approaches.
What’s next in your research?
In our future research we wish to go beyond the modelling of small atmospheric molecular clusters and into the modeling of realistic sized freshly nucleated particles. This is an enormous leap forward in the field but will most likely also give rise to many obstacles as we might need to model up to hundreds of molecules. Our first course of action is to attempt to figure out the composition of freshly nucleated particles and how they interact with their environment.
There lie many challenges ahead, as the usual quantum chemical methods used to model small clusters are simply too computationally expensive to apply to freshly nucleated particles. However, I am eager to get started on this new adventure into unknown territory.
What physical chemistry problems are you hoping to see get solved in the next decade?
Atmospheric aerosol particles remain the largest uncertainty in global climate estimation. In order to truly reduce this uncertainty molecular-level data are required. My hope is that within ten years, we can model realistic sized aerosol particles using state of the art QC+ML methods. Obtaining molecular level data on aerosol particle processes, such as formation, growth, chemical reactions at the surface or inside and implementing such processes into models will effectively push the field to a completely new level.
While there is a long way there, I sincerely hope that we will see more molecular modelling of aerosol particles in the future.
Explore articles published by Jonas Elm in ACS Publications journals:
Krupa Ramasesha – The Journal of Physical Chemistry B Recipient
The Journal of Physical Chemistry B award honors an investigator in the areas of biophysics, biomaterials, liquids, and soft matter.
Krupa Ramasesha is a Staff Scientist at the Combustion Research Facility at Sandia National Laboratories in Livermore, California. She is leading a research program that investigates ultrafast photo-induced energy and charge transfer dynamics in the gas and condensed phases, employing ultrafast electronic and vibrational spectroscopies as well as ultrafast electron diffraction. She received a B.S. in Chemistry from the University of Wisconsin-Madison, where she worked with Fleming Crim exploring solution-phase radical reactions and photoisomerization dynamics via time-resolved electronic spectroscopy. Krupa earned her Ph.D. from the Massachusetts Institute of Technology working with Andrei Tokmakoff to investigate hydrogen bonding dynamics in water and aqueous proton transfer dynamics using ultrafast broadband multidimensional infrared spectroscopy. Subsequently, she worked with Stephen Leone and Daniel Neumark at the University of California, Berkeley, as a postdoctoral fellow studying attosecond electron dynamics in semiconductors using soft X-ray core-level spectroscopy.
Prior to starting as a staff scientist, Krupa worked with David Osborn at Sandia, investigating chemical reaction kinetics relevant to combustion and atmospheric chemistry employing synchrotron-based photoionization mass spectrometry.
What do you consider to be the most important advancement in physical chemistry in the past five years?
The past five years have seen accelerated deployment of ultrafast core-level spectroscopies and ultrafast diffraction techniques, both on the lab-scale and in large-scale facilities, providing unprecedented insights into temporally and spatially resolved electronic and structural dynamics and their complex interplay. It has been particularly exciting to see these approaches being applied to study textbook examples of molecular transformations and interactions, providing a deeper understanding of widely taught concepts surrounding the nature of chemical bonding. These experimental breakthroughs have also presented new opportunities for benchmarking and constraining theoretical methods for systems ranging in chemical complexity.
What advances has your lab made in the past five years?
The goal of my research program is to understand the ultrafast chemical dynamics governing energy conversion in photo-excited gas phase and condensed phase systems. Over the past five years, my lab has investigated vibrational, structural, and electronic dynamics governing two- to many-body photodissociation of transition metal carbonyl photocatalysts, disulfides, and organic halides in the gas phase.
These studies have shed light on the excited state dissociation mechanisms and the intricate non-adiabatic dynamics that underpin them. In the condensed phase, we have studied ultrafast vibrational energy transfer in molecular solids such as energetic materials, revealing both fast and surprisingly slow vibrational dynamics that contribute to the chemical processes preceding initiation. My lab employs a suite of nonlinear spectroscopy techniques, such as ultrafast broadband infrared spectroscopy, ultrafast core-level spectroscopy, and ultrafast electron diffraction, with the objective of developing a comprehensive picture of the chemical dynamics.
What’s next in your research?
The research areas I plan to explore over the coming years include ultrafast excited state dynamics of open-shell species as well as intramolecular, intermolecular, and interfacial photoinduced charge transfer dynamics, with the goal of both understanding and perhaps controlling these processes. To this end, we will continue to employ and develop nonlinear spectroscopic techniques spanning the infrared to X-ray regimes, coupled with the application of high-level electronic structure theory through collaborations.
What physical chemistry problems are you hoping to see get solved in the next decade?
Despite its applicability to most all photophysical and photochemical processes, developing accurate descriptions of excited state chemical dynamics has remained an enduring challenge in physical chemistry. This is particularly the case for excited state charge transfer, where it is well known that the dynamics are not only complicated by non-adiabatic effects but also by strong electron correlation, and, especially in the case of organometallics, relativistic effects.
I am hoping to see, and expect to contribute to, scientific advances that enable predictive understanding of charge transfer dynamics through systematic investigations starting from isolated molecules and building up to more complex systems. Such investigations will require the use of incisive and diverse spectroscopic tools, in conjunction with theory, to monitor both electronic and structural dynamics underlying excited state charge transfer.
Explore articles published by Krupa Ramasesha in ACS Publications journals.
Joel Yuen-Zhou – The Journal of Physical Chemistry C Recipient
Joel Yuen-Zhou graduated with B.Sc. in Chemistry and B.Sc. in Mathematics in 2007 from MIT, and a Ph.D. in Chemical Physics in 2012 from Harvard, where he studied nonlinear optical spectroscopies from the viewpoint of quantum information processing under the supervision of Alán Aspuru Guzik. In 2013-2015, he held the Robert J. Silbey Postdoctoral fellowship at the Center for Excitonics at MIT, where he explored aspects of topological protection in excitonic and polaritonic systems.
Since 2015, he has been a faculty member at the Department of Chemistry and Biochemistry of UCSD, becoming Associate professor with tenure in 2020. His research encompasses the theoretical study of unconventional regimes of molecular light-matter interactions including the strong and ultrastrong coupling regimes. He has been a recipient of the NSF CAREER (2017), DOE Early Career (2018), ACS Open Eye (2019), Sloan Fellowship (2021), Camile Dreyfus Teacher Scholar (2021) awards, and a finalist in the Blavatnik National Awards for Young Scientists (2020).
What do you consider to be the most important advancement in physical chemistry in the past five years?
I am not sure I can pinpoint a single most important advancement. I see a number of exciting frontiers emerging (several of which I have not been involved with), including applications of machine learning to computational chemistry or the discovery of unifying principles to understand fluctuations in nonequilibrium thermodynamics, to mention a few.
Closer to my area of research, I regard the experimental demonstration of unconventional regimes of light-matter interaction at room-temperature, such as strong and ultrastrong coupling of optical micro- and nanocavities to molecular systems, and its use towards the control of physicochemical properties of molecular materials, as one of the most important advancements in physical chemistry.
What advances has your lab made in the past five years?
My research group has provided theoretical and computational tools to describe the mechanisms whereby condensed phase chemical dynamics and molecular spectroscopy can be affected by strong light-matter coupling in optical microcavities. Some of the work we are most excited about are on polariton-assisted photophysics, energy transfer, and remote chemistry, the harnessing of novel optical nonlinearities mediated by cavities, and cavity reaction-rate theories.
Besides polariton chemistry, my group is also broadly interested in other unconventional light-matter interactions, such as Floquet engineering and laser dressing. These ideas have been popularized in the solid-state community, but less so in the molecular materials one. For instance, we are very excited about recent work where we show that topological frequency conversion can distinguish between two types of molecular enantiomers.
What’s next in your research?
Polariton condensates (nonequilibrium analogues of Bose-Einstein condensates) are states featuring a macroscopic number of excitations in a single polariton mode. They have been routinely demonstrated within the last decade in a variety of organic dye microcavities. Surprisingly, their potential as agents that undergo chemical change had not been explored until recently, where we demonstrated that they offer unique energy redistribution processes that cannot be found in bare molecular systems excited with laser light.
I believe that chemistry with polariton condensates is an exciting frontier in physical chemistry that could challenge well-established paradigms in chemical physics, and we are currently working on elucidating their fundamental physicochemical properties.
What physical chemistry problems are you hoping to see get solved in the next decade?
A great mystery in the field is vibropolaritonic chemistry, where chemical reaction rates are experimentally reported to be modified under collective vibrational strong coupling. At present, theories that acknowledge the collectivity of the light-matter coupling cannot explain changes in adiabatic reaction rates within the framework of equilibrium transition-state theory. I believe it is of paramount importance to resolve this experiment/theory inconsistency.
Explore articles published by Joel Yuen-Zhou in ACS Publications journals.