Many of us are fascinated by dinosaur fossils, and it’s truly mind-blowing when these imprints of ancient life still contain organic matter. But how are these proteins lasting for millions of years without degrading?
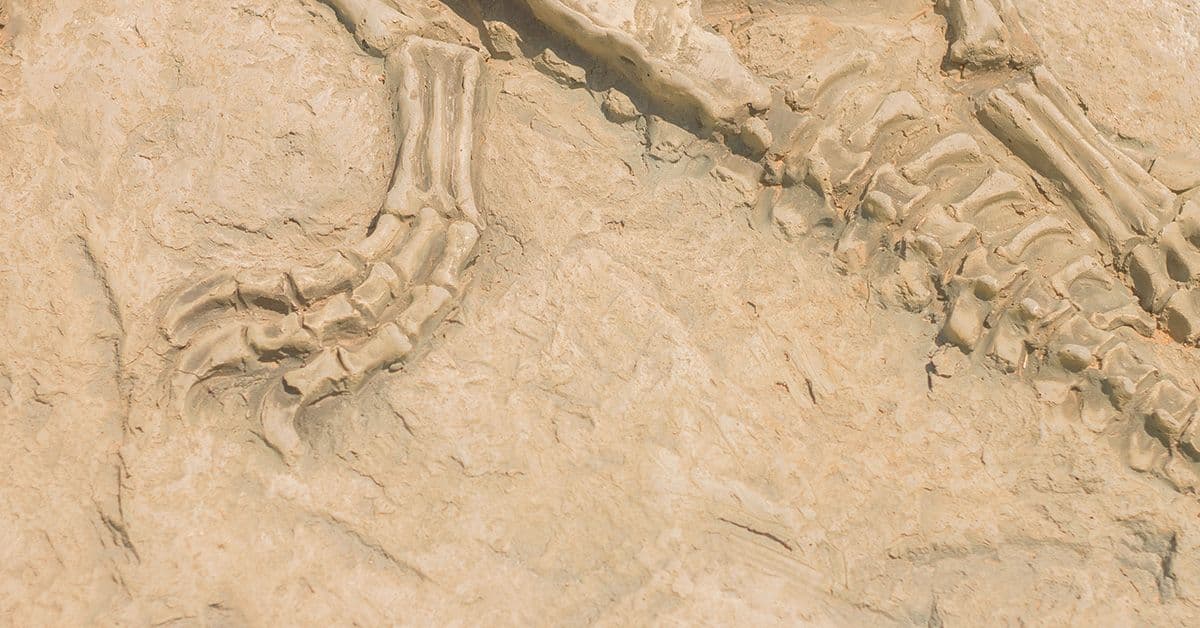
Contrary to popular film lore, the oldest DNA extracted from a fossil is a mere 1.2 million years old—coming not from a dinosaur, but from a Siberian mammoth.1 Even modern environmental DNA techniques can take us back only 2 million years.2 So although preserved ancient proteins could provide critical information, it has traditionally been assumed that they are outside the reach of dinosaur paleontology. But there are other organic traces—notably collagen, the most abundant animal protein found in skin, bone, and connective tissue. Collagen consists of three polypeptide chains that wind around each other to form a triple helix, which is crucial for its strength and stability. Each of these chains is made up of repeating sequences of amino acids. When exposed to water, the peptide bonds that connect amino acids are usually broken down in hydrolysis—with a half-life of around 500 years in a neutral aqueous solution. However, collagen has been found intact in some truly ancient bones, with fragments extracted from the remains of creatures dead for 195 million years.3 Various hypotheses have been proposed to explain this resistance of peptide bonds in collagen, but until now a physical and chemical basis was lacking.
In a new study published in ACS Central Science, a team from MIT report on the behavior of small-molecule mimics of collagen peptides using experimental and computational methods.4 In particular, they studied the interactions between acyl groups, which each contain a carbon atom double bonded to an oxygen atom. These interactions occur between the lone pair of electrons (n) on the oxygen atom of one peptide bond and the antibonding orbital (π*) of the carbonyl group in an adjacent peptide bond. This electron sharing helps stabilize the peptide bond, making it less likely to be attacked by water molecules. Based on this, the group hypothesized that engagement of a π* orbital in an n→π* interaction could be what protects peptide bonds from hydrolysis. Using a combination of molecular torsion balance and computational tools, they found that each acyl group partially shares its electrons with a neighboring acyl group. These results suggest that such interactions protect every peptide bond in a collagen triple helix from hydrolysis, allowing the structure to stay intact. The authors note that such insights into the stability mechanisms of collagen can inform the design of more long-lasting biomaterials.
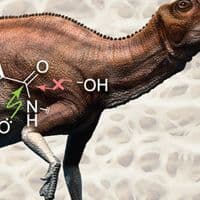
Pauli Exclusion by n→π* Interactions: Implications for Paleobiology
DOI: 10.1021/acscentsci.4c00971
Other research has shown that peptides can be repeatedly detected and validated from fossil tissues many millions of years old, and with new techniques come new insights. For example, updated extraction methodology, high-resolution mass spectrometry, and bioinformatics have been used to analyze a Brachylophosaurus canadensis specimen from which collagen peptides were recovered in 2009—a date which perhaps feels like yesterday but is already ancient history in the field of paleoproteomics.5
Just eight years later, new techniques were able to recover eight peptide sequences—two of which were identical to those recovered in the original research, and six new peptides not previously identified. Incredibly, these new peptides changed the phylogenetic understanding of this ancient animal, placing it more closely to basal birds than crocodylians. In addition to showcasing the value of peptide samples from millions of years ago in classifying and understanding fossils, the study also demonstrates the importance of re-examining specimens with updated methods and instrumentation as it becomes available.
With teams making these valuable findings, paleoproteomics—the study of ancient proteins—is becoming an important multidisciplinary field at the intersection of molecular biology, paleontology, archaeology, paleoecology, and history. A great piece in Chemical Reviews provides an overview of the history of the field, a summary of the major methods and applications currently in use, and a critical evaluation of challenges.6 Another perspective in the Journal of Proteome Research focuses on future methods for and applications of deep-time proteomics.7 Decontamination and authentication remain important aspects, but with careful protocols new techniques can be applied to an increasingly broad range of taxa, depositional environments, and age ranges.
Far from being simply about the history of proteins and extinct creatures, concerted, community-wide efforts to fully evaluate and characterize molecular content from fossils are expected to yield new approaches to age-old problems, from evolution to human health. Additionally, what we learn from the stability of ancient proteins could help guide the design of other exceptionally long-lived materials for applications such as tissue engineering and regenerative medicine.
Explore Related Research Published in ACS Journals
Conformational Stability of Collagen Relies on a Stereoelectronic Effect
Lynn E. Bretscher, Cara L. Jenkins, Kimberly M. Taylor, Michele L. DeRider, and Ronald T. Raines
DOI: 10.1021/ja005542v
Conformational Preference and Cis−Trans Isomerization of 4(R)-Substituted Proline Residues
Il Keun Song and Young Kee Kang
DOI: 10.1021/jp054351h
A Crystal Structure of an Oligoproline PPII-Helix, at Last
Patrick Wilhelm, Bartosz Lewandowski, Nils Trapp, and Helma Wennemers*
DOI: 10.1021/ja507405j
The Yin and Yang of How N-Terminal Acyl Caps Affect Collagen Triple Helices
Tomas Fiala, Rahel Heeb, Luca Vigliotti, and Helma Wennemers*
DOI: 10.1021/acs.biomac.3c00241
References
- Price, M. Mammoth molars yield the oldest DNA ever sequenced. Science.org, 17 Feb 2021.
- Kjær, K.H., Winther Pedersen, M., De Sanctis, B. et al. A 2-million-year-old ecosystem in Greenland uncovered by environmental DNA. Nature 612, 283–291 (2022).
- Lee, Y.-C. et al. Evidence of preserved collagen in an Early Jurassic sauropodomorph dinosaur revealed by synchrotron FTIR microspectroscopy. Nat. Commun. 8, 14220 (2017).
- Yang, J. et al. Pauli Exclusion by n→π* Interactions: Implications for Paleobiology. ACS Cent. Sci. 2024.
- Schroeter, E.R. et al. Expansion for the Brachylophosaurus canadensis Collagen I Sequence and Additional Evidence of the Preservation of Cretaceous Protein. J. Proteome Res. 2017, 16, 2, 920–93.
- Warinner, C. et al. Paleoproteomics. Chem. Rev. 2022, 122, 16, 13401–13446.
- Schroeter, E.R. et al. Deep Time Paleoproteomics: Looking Forward. J. Proteome Res. 2022, 21, 1, 9–19.