Our bodies are complicated but ordered systems in a constant state of change, with a dance happening inside each and every cell from the moment of our conception to our death. Now, researchers are putting their dancing shoes on to learn the steps for vesicle self-assembly.
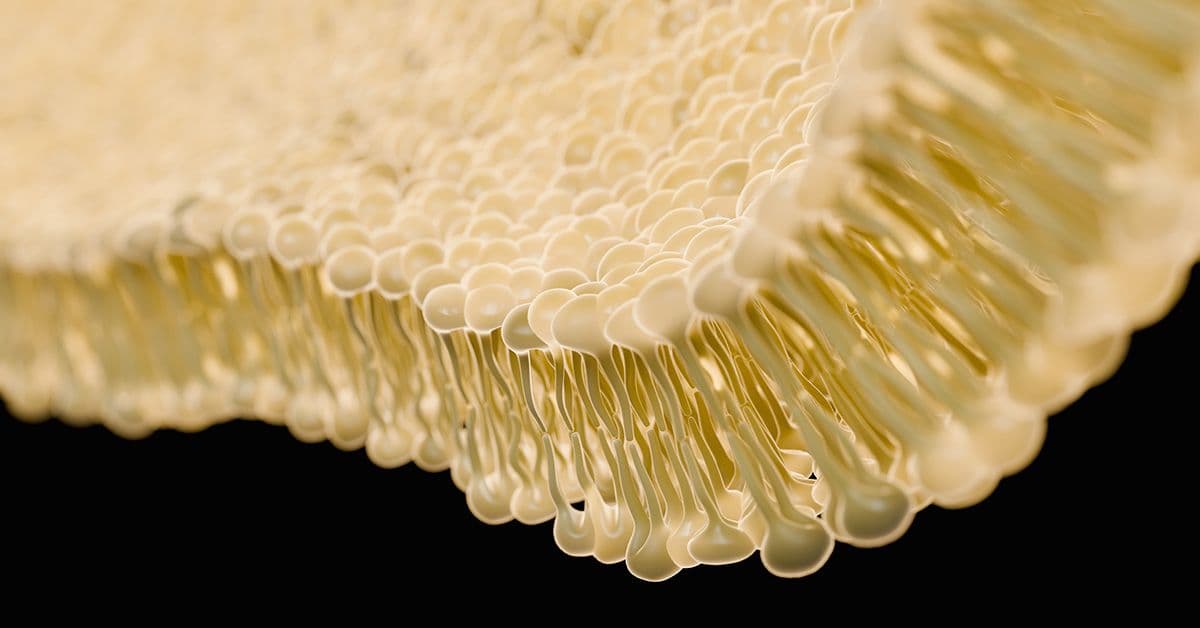
Dance is an art, but anyone who has been to the ballet will know it is also a precise science. Choreography dictates that the dancers land at exactly the right spot on the right beat—for the beauty of the show and the story, but also for their safety. Nobody wants to see the prima ballerina commit herself to the air before her partner is ready to catch her. Within biological systems, there is also a constant dance at play, with molecules made or broken down, receptors activated, and a whole host of chemicals moved from one place to another. A constant flux is happening within our bodies at all times as trillions of cells perform vast numbers of simultaneous chemical reactions.
A top-billed cast member in this dance is the lipid bilayer, a key building block of cellular membranes and vesicles. These bilayers, known for their self-assembly, can transport waste and other chemicals around the cell interior, as well as assist with cell division, fertilization, and viral entry.1 You may remember drawing these in high school biology, with lipids neatly aligned like a double row of old-fashioned clothespins. The self-assembly comes about due to the amphipathic nature of lipid molecules, which have both hydrophobic and hydrophilic regions.
Lipid bilayers can be incredibly versatile and adopt an array of diverse shapes; however, their dynamic behavior and intricate shapes have been difficult to study experimentally due to their complexity and the limitations of observation techniques. But now, researchers in Argentina are exploring these natural phenomena a bit more closely to see how topography and configuration evolves under different conditions.2
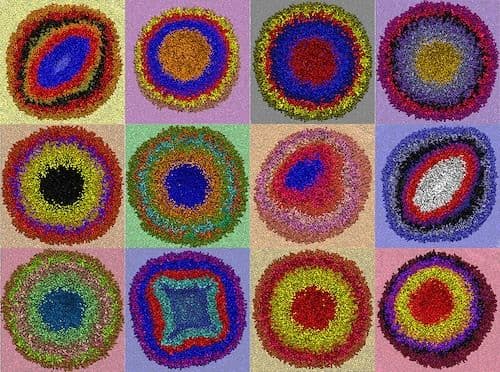
The study, published in ACS Nano, investigates the complex dynamics of lipid vesicles, uncovering a range of unexpected geometric patterns and shapes they can adopt. The identified five key structural patterns that recur across different conditions, including elongated or flattened shapes (oblates and prolates), doughnut-like structures (toroids), cup shapes (stomatocytes), and spheroids. These findings enhance our understanding of how these vesicles self-organize, offering valuable insights into their behavior within biological systems and potentially informing the design of new biomimetic materials.
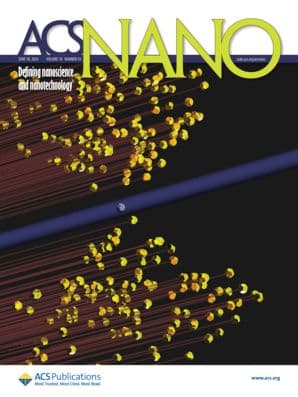
The Secret Ballet Inside Multivesicular Bodies
DOI: 10.1021/acsnano.4c01590
Limit shapes of confined multivesicular bodies have previously been predicted, but only a few have been observed experimentally before. In 2012, research published in the Journal of the American Chemical Society describes the entrapment of metal nanoparticles in polymers.3 The cup-like stomatocyte morphology was again identified here and was found to be an ideal shape to physically trap nanoparticles, demonstrating clear potential use in heterogeneous catalysis and drug delivery.
The lack of experimental observation in this field is partly due to the inherent complexity of the systems being studied. This new study from Luis S. Mayorga and Diego Masone relied on two key previous advances: first, a novel algorithm published in 2021 used to help track individual lipid leaflets, allowing for easy and quick detection of topological changes at the mesoscale;4 and second, the latest version of the Martini model—Martini 3—which offers significant improvements in interaction balance, molecular packing, and inclusion of new bead types and sizes.5
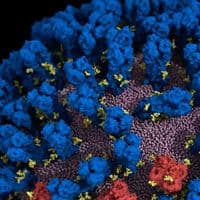
How the Flu Moves
The authors report that understanding the self-assembly of lipid vesicles paves the way for designing bio-inspired artificial transporters and materials with self-assembly properties. These vesicles could revolutionize drug delivery systems, enabling more efficient and targeted therapies. Moreover, the insights gained from this study could inspire interdisciplinary innovations, bridging the gap between synthetic and biological systems. By harnessing the innate dynamism of lipid bilayers, scientists can create materials that mimic the complex behaviors of living organisms, opening new possibilities in biotechnology and nanotechnology.
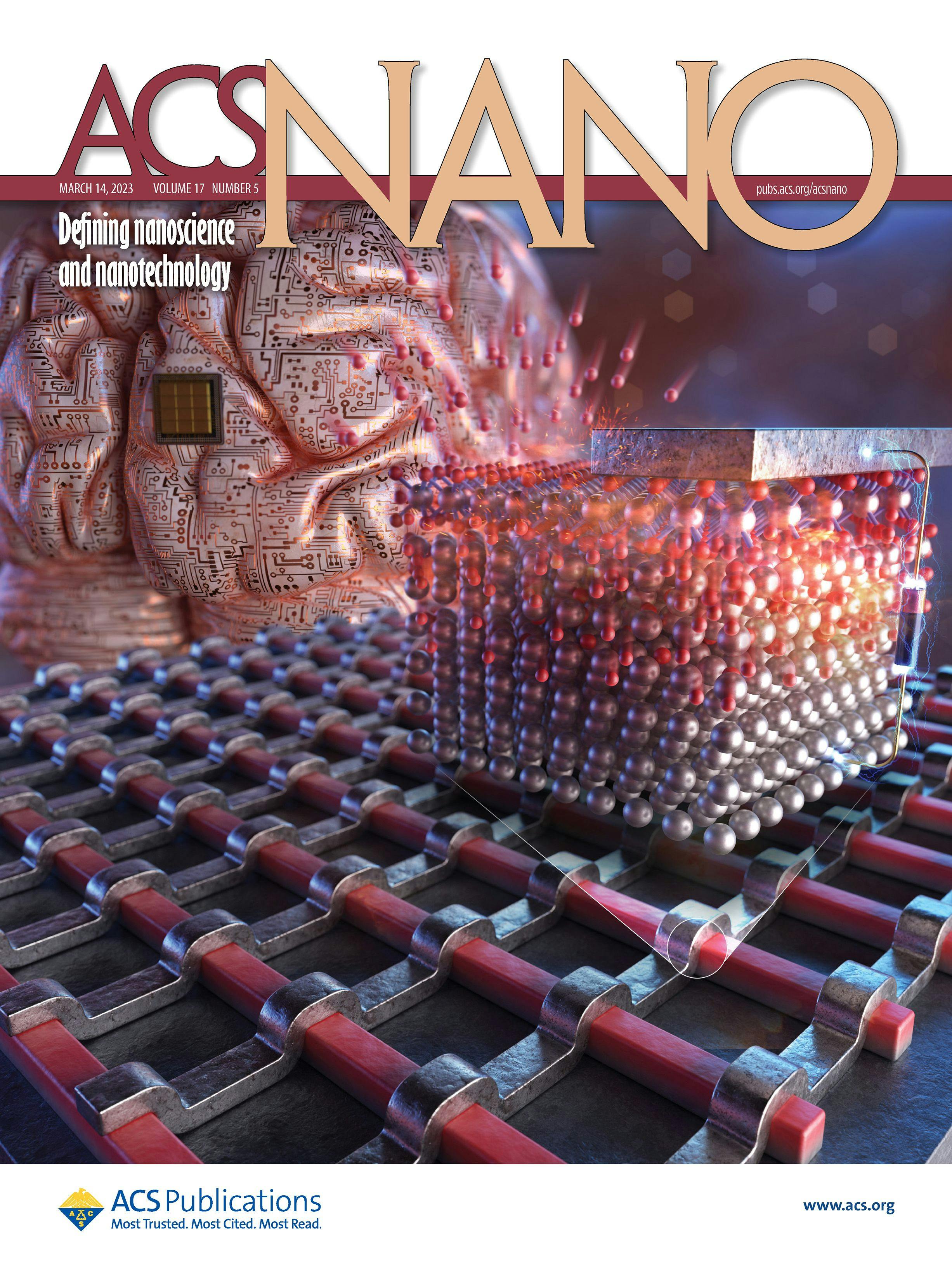
Stay Connected with ACS Nano
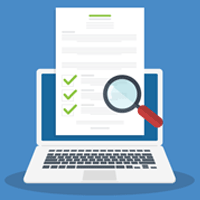
Submit Your Research
References
- Shin, W. et al. Visualization of Membrane Pore in Live Cells Reveals a Dynamic-Pore Theory Governing Fusion and Endocytosis. Cell 2018, 173, 934– 945.e12.
- Mayorga, L. S. and Masone, D. The Secret Ballet Inside Multivesicular Bodies. ACS Nano 2024, 18, 24, 15651–15660.
- Wilson, D. A. et al. Entrapment of Metal Nanoparticles in Polymer Stomatocytes. J. Am. Chem. Soc. 2012, 134, 24, 9894–9897.
- Bruininks, B. M. H. et al. Sequential Voxel-Based Leaflet Segmentation of Complex Lipid Morphologies. J. Chem. Theory Comput. 2021, 17, 12, 7873–7885.
- Borges-Araújo, L. et al. Martini 3 Coarse-Grained Force Field for Cholesterol. J. Chem. Theory Comput. 2023, 19, 20, 7387–7404.