Systems for electrochemical energy storage and conversion include batteries, fuel cells, and electrochemical capacitors.[1] Recent advances in lithium battery technology have gained attention for their potential to influence the evolution and adoption of electric vehicles. However, over the past decade, some leading names in the field of electric vehicles (Elon Musk, for one) have predicted […]
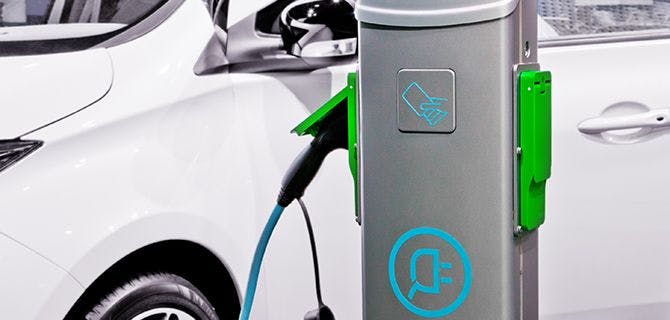
Systems for electrochemical energy storage and conversion include batteries, fuel cells, and electrochemical capacitors.[1] Recent advances in lithium battery technology have gained attention for their potential to influence the evolution and adoption of electric vehicles. However, over the past decade, some leading names in the field of electric vehicles (Elon Musk, for one) have predicted that the supercapacitor could surpass batteries as the key to their advancement.[2] Supercapacitors use aqueous membranes to store energy in an electrical field – unlike batteries, which use a chemical store. Some of the advantages to supercapacitors over batteries are faster charging times and increased life cycle, plus a move away from reliance on rare elements such as lithium.[3]
Despite their different characteristics, supercapacitors have a similar configuration to traditional batteries – including an anode, cathode, and electrolyte.[4] But rather than relying on redox reactions as in a traditional battery cell, in the supercapacitor energy is delivered by the orientation of ions at the electrolyte interface, where electrical double layers (EDLs) are formed and released – generating parallel movement of electrons in an external wire.[1] The characteristics of the particular EDL depend on the surface structure of the electrode, electrolyte composition, and the potential field between charges at the interface.[1] Despite their intriguing potential supercapacitors have found utility in only a few niche products, since poor ion mobility within the conducting polymer has often hindered performance [3] – until now.
An Unexpected Discovery
In the 1970s, of scientists at Superdielectrics Ltd were working on developing a new material for contact lenses. This lens was formed from a transparent, flexible hydrocarbon polymer that held water like a sponge. 20 years later, the same team found that the same cross-linked, gel-matrix polymer mesh could be made to conduct electricity by adding different chemicals. Researchers investigated the tech as a way to connect prostheses to the nervous system. During testing, it became apparent that they had created a material with capacitance up to 10,000 times greater than existing conductors. Scientists predict this chance finding could deliver energy densities of up to 180 Wh/kg – 18-times greater than the best currently available supercapacitors.[5],[6] Researchers’ focus has switched to trialing fuel cells that use electrodes made from foil coated with a thin layer of the polymer.[6]
By using small, single-layer cells charged to 1.5 volts for 2–5 minutes the researchers were able to run a small fan; a three-cell stack rapidly charged to 5 volts was able to operate an LED.[7] If commercial production of these high-capacity supercapacitors becomes possible, it could revolutionize the way devices store and use electricity. On the level of personal electronics, polymer gels made with green tea extracts could form the basis of a supercapacitor that could power comfortable wearable tech such as fitness trackers or heartrate monitors.[8] On a larger scale, supercapacitors could allow the grid to accept and release charge quickly, solving many demand problems and helping to balance out the intermit input of renewables.[6]
In its inaugural issue in February 2018, ACS Applied Energy Materials published research on supercapacitors utilizing macroporous graphene,[9],[10] multilayer films comprised of dielectric polymers,[11] and even high-performance supercapacitors using carbon derived from biowaste.[12] This new title will continue to monitor the rise of supercapacitors and chart new technological innovations that bring us closer to achieving sustainable energy goals.
[1] Winter. What Are Batteries, Fuel Cells, and Supercapacitors? Chem Rev 2004;104(10):4245–4270.
[2] Elon Musk Says Super-Capacitors Not Batteries, Will Be Breakthrough For EVs. Available at: https://techcrunch.com/2011/03/25/elon-musk-says-super-capacitors-not-batteries-will-be-breakthrough-for-evs/ (accessed March 2018).
[3] Fong et al. Semi-Interpenetrating Polymer Networks for Enhanced Supercapacitor Electrodes. ACS Energy Lett 2017;2(9):2014–2020
[4] Zho. Battery-Supercapacitor Hybrid Devices: Recent Progress and Future Prospects. Advanced Science 2017;4(7):1600539.
[5] Alternative to traditional batteries moves a step closer after exciting progress in supercapacitor technology. Available at: http://www.bris.ac.uk/news/2018/february/supercapacitor.html (accessed March 2018).
[6] UK supercapacitor results suggest challenge to battery technologies. Available at: https://www.theengineer.co.uk/supercapacitors-battery-technologies/ (accessed March 2018).
[7] Press release, 26th February 2018. Available at: http://www.supercapacitormaterials.com/ (accessed March 2018).
[8] Das et al. Elastic Compressible Energy Storage Devices from Ice Templated Polymer Gels treated with Polyphenols. J Phys Chem C 2017;121(6):3270–3278.
[9] Down & Banks. Freestanding Three-Dimensional Graphene Macroporous Supercapacitor. ACS Appl Energy Mater 2018;1(2):891–899.
[10] Down et al. Fabrication of Graphene Oxide Supercapacitor Devices. ACS Appl Energy Mater 2018;1(2):707–714.
[11] Huang et al. Reduction of Ionic Conduction Loss in Multilayer Dielectric Films by Immobilizing Impurity Ions in High Glass Transition Temperature Polymer Layers. ACS Appl Energy Mater 2018;1 (2):775–782.
[12] Yang et al. Biowaste-Derived Three-Dimensional Porous Network Carbon and Bioseparator for High-Performance Asymmetric Supercapacitor. ACS Appl Energy Mater 2018:1(2);616–622.